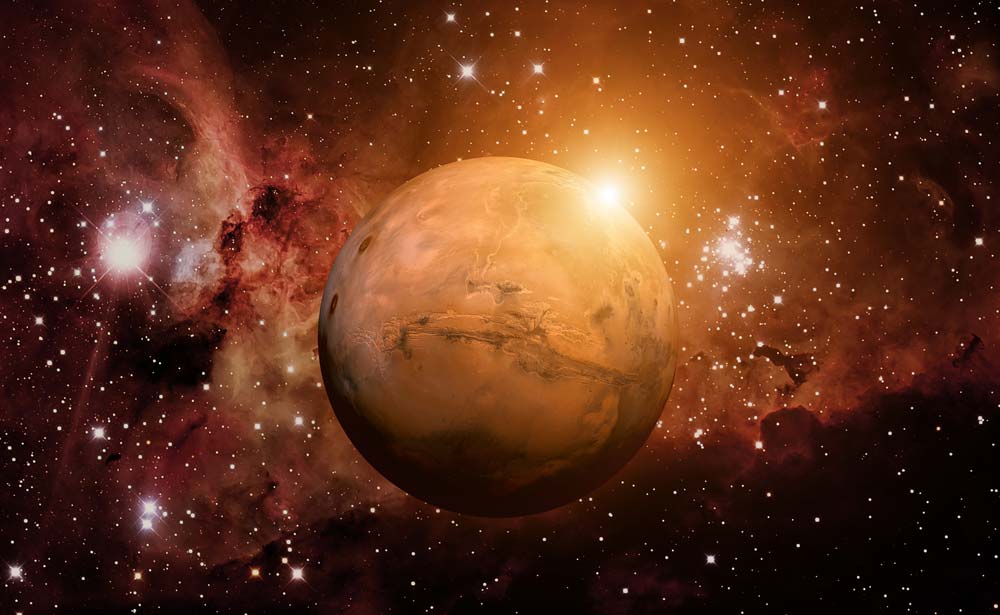
Life on Earth exists in a wide variety of environments, including so-called extremophiles discovered only about 50 years ago. By adopting a macroscopic view on the formation of life, we can study which planets and planetary environments are favourable for the origin of life, whether those are stable and, if not, whether life can adapt to them on the timescales on which they change.
In particular, the diversity of Earth-like planets that could be favourable for life will be studied. What ranges of planet size, temperature, external radiation, and surface properties would still accommodate life as we know it? To which extent are global geophysical characteristics – like the presence of liquid water, subsurface water, a magnetic field, plate tectonics, a moon – necessary pre-conditions or boundary conditions for life? What is the required orbital and dynamical stability of a planetary system so that life can develop? Can we quantify the importance, spatial distribution and timing of the delivery of building blocks of life (at the elemental and/or molecular level) to young planets? An important aspect for the formation, evolution and survival of life is how the atmospheric structure and its composition evolve from the primordial to the secondary atmosphere including global processes such as outgassing of the mantle, volatile losses, and external delivery by asteroids, comets, and dust.
2. Defining properties and synthesis of Life, from the molecular to the biosphere level
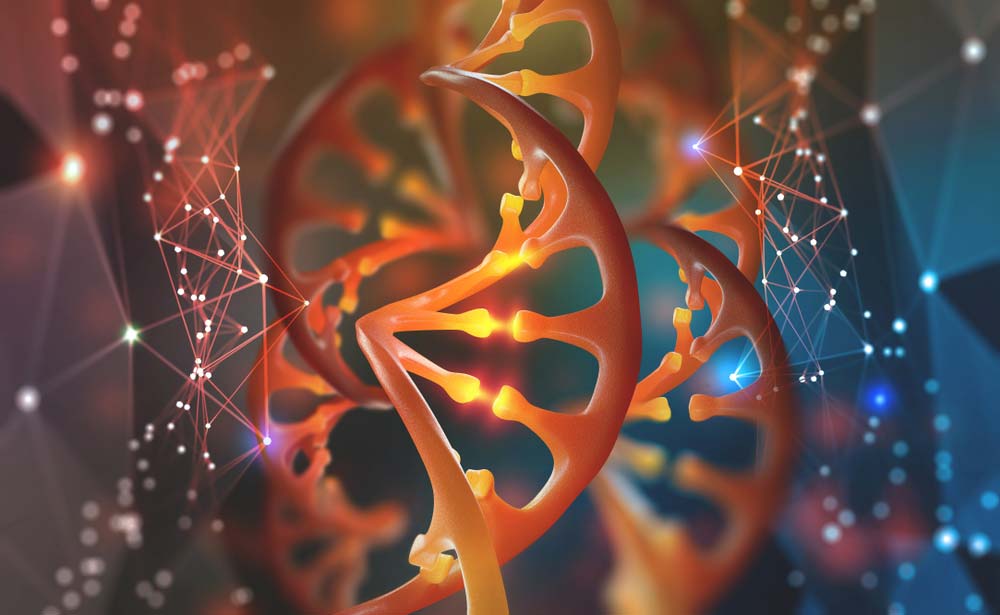
While lipids, proteins and nucleic acids are regarded as non-living materials, their assembly results in hierarchically ordered structures (such as bacteria or eukaryotic cells), which are clearly alive. How does nature tackle the grand complex challenge to order the many copies of all the different lipids, proteins and nucleic acid molecules into a functional, active and living structure? How is it assured that all the different cells in organs and organisms are at the right place after a complex cell division and differentiation process?
Complexity also plays an important role on larger length scales, where organisms interact with each other, and where finally the set of ecosystems make up our constantly evolving biosphere. What is the basis of this, what are the sources and mechanisms of variability and diversity? How important are genetic factors compared to nongenetic ones, is selective retention of variants a prerequisite for evolution or an outcome of it?
Here we will address such questions by studying existing systems at different levels, for instance using advanced light and force microscopy approaches zooming in on individual molecules, observational approaches for zooming out to larger length scales and mathematical modelling to describe and link the different levels. In addition, new systems will be created such as artificial (sub)cellular structures. For the latter, constructing a synthetic form of life constitutes a huge challenge. This may be approached by the bottom-up construction of compartments with life-like features, by gathering all needed components and trying to assemble them in the right order, using either naturally existing biomolecules, or components synthesised ab initio. Also at the scale of the biosphere, there is a need for assembly. Can one build a biosphere on up to now inhabitable planets? What are the prerequisites for this?
3. Modelling, predicting and steering of Life
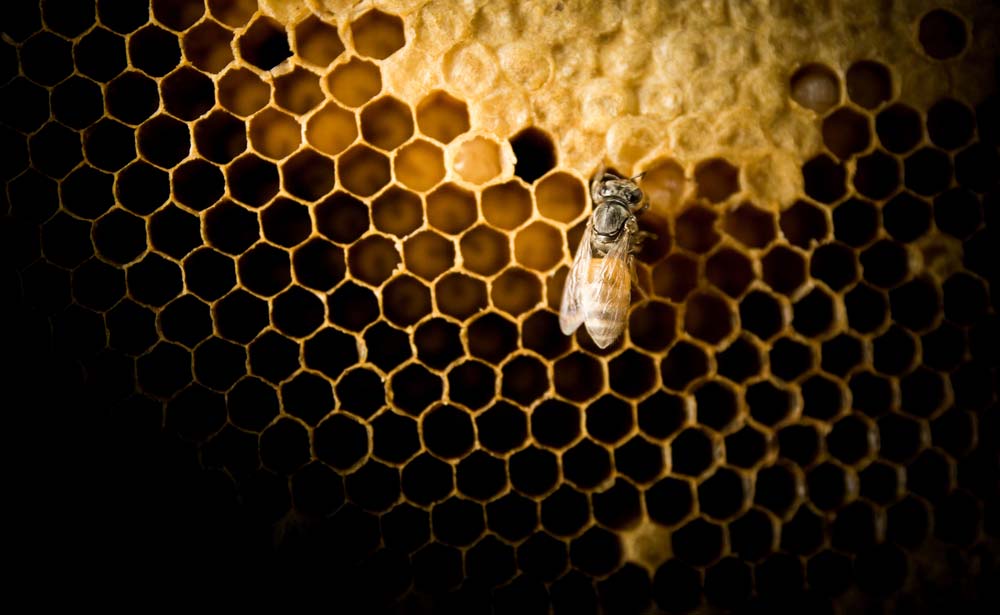
All known living systems share a number of basal features: (a) they are complex, containing large numbers of interacting components; (b) they are hierarchically organized (from molecules and their assemblies to cell organelles, to cells, to tissues, to organs, to organisms, to populations, to communities, to ecosystems); (c) they are the product of adaptive processes like natural selection; and (d) they are responsive in that they adapt their state and behaviour to changes in local conditions.
It is a major challenge to understand the functioning of such complex adaptive systems, to predict their responses to changing conditions, and to steer these systems in a desired direction, e.g. by increasing their capacity to adapt to human-induced environmental change. Meeting this challenge comes, for the first time in human history, within reach.
As a result of the ‘omics’ revolution, biological data of unprecedented scope, depth and detail have become available. On the computational side, major technological advances in hardware and software development make it possible to develop new simulation paradigms, which take a systems-level perspective, thus integrating data from a variety of sources and allowing to make detailed testable predictions. We are in an ideal position to develop such ‘new-generation’ models (and the tools to analyse them), because the required technical know-how and infrastructure is available, and our multidisciplinary consortium comprises expertise on all levels of chemical and biological organisation.
Such modelling is relevant to major scientific questions as well as societal concerns: to map the scope and limitations of the adaptive capacity of organisms to cope with climate change; to predict the evolution of pathogens in order to design effective countermeasures; to develop strategies against multidrug resistance; to design ‘sustainable landscapes’ satisfying human needs (e.g. food production) yet minimizing the long-term loss of biodiversity. Our know-how to develop a new generation of ‘evolutionary engineering’ tools will also be used, allowing to tackle complex engineering problems such as designing ‘autonomous’ machines.
4. Distribution of Life across the universe
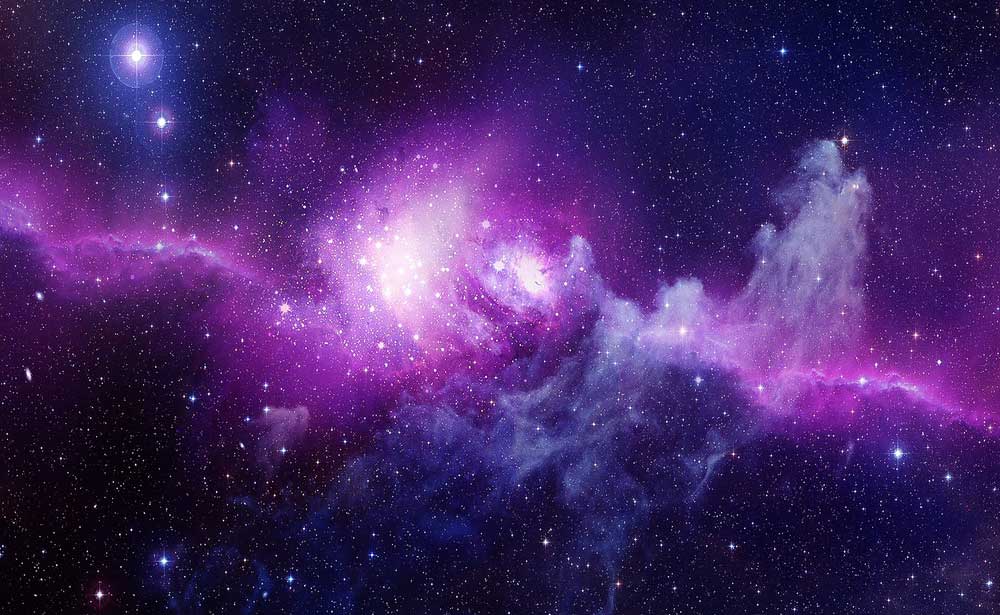
Both researchers and the general public are fascinated by searches for signs of life outside the Earth. Within our Solar system, in situ methods may be used for such searches, while for exoplanetary systems, remote sensing is the only viable option. The success of either approach critically depends on a good understanding of what unambiguous signs of life would be.
While we are limited to terrestrial examples, our programme aims at exploring the widest possible range of habitable environments that the Earth offers, and extrapolating these results to likely conditions on extrasolar planets. The aim is a clear set of selection criteria for likely habitable planets, based on physical parameters such as mass, temperature, and magnetic field. An essential part of this research area is the identification and validation of potential biosignatures. Within our Solar system, these may be particular compositions and morphologies of sediments and rocks; on exoplanets, the prime goal are atmospheric gases. In both cases, risk assessment is critical to avoid false positives and negatives. Besides the validity of biomarkers, we will study their visibility to current and future instrumentation. In fact, searches for biomarkers may well drive the design of future missions. oLife aims to establish specifications for future instrumentation to maximize the chances for successful search for life beyond the Earth.